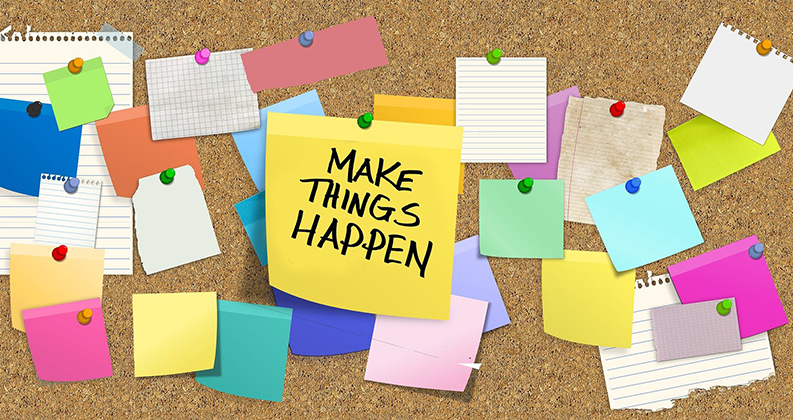
Regulatory changes, cost pressures and our own human behavior may make product launches difficult. These nine rules can ensure that your next project is successful.
#1) Think Backwards: Design Output > Design Input > User Needs
My #1 rule for successful development of orthopedic products is that every design output on drawings and in specifications, every feature, every note, must be tied back to a user need. User needs are not limited to the needs of the patient or surgeon. Often user needs are driven by business needs. Marketing wants a molded handle to differentiate from competitive products. Legal has restricted use of a desired feature due to IP issues resulting in a design output choice. The most critical process in developing successful orthopedic products is a complete and accurate definition of user needs, and the translation of these needs into design features.
#2) Set Cost Targets
The orthopedic industry has changed and continues to change. Market prices are experiencing downward pressure, creating revenue headwinds. Large orthopedic companies are finding it difficult to grow revenue, and thus trying to maintain bottom line profits by cost cutting throughout the entire organization. Finding a blockbuster $100 million product in this mature market is becoming extremely rare. The cost to launch a new product that will cover the full market demand can be staggering. It’s critical to have clear financial models with cost targets for both sellable products and capital investment for instrument sets. Regular reviews/updates of the financial models should be conducted early in the development process.
#3) Define Special Process Requirements
The regulations are clear, “where the results of a process cannot be fully verified by subsequent inspection and test, the process shall be validated…”1 A process where the results cannot be validated by a nondestructive inspection is typically referred to as a special process. Special processes, therefore, must be validated.
Product engineers often have features that require special processes or list special processes on the engineering drawings. What’s often missing is a definition of the “required results” of the process. For example, a design has a requirement for welding. The engineer puts a weld symbol pointing to the weld joint and states that the weld must be validated. This raises the question, validated to what? A strength requirement? A requirement for radiographic or ultrasonic inspection? For corrosion resistance? If the result of the process is not defined, the process cannot be validated to ensure that design requirements are met. The design engineer may say it’s up to manufacturing to validate the process. This is true, but manufacturing cannot validate a process if the process results are not clearly defined.
Passivation is typically considered as a special process used to create a continuous passive surface layer by removal of exogenous iron. Passivation can also increase the corrosion resistance of stainless steel by depleting the surface of iron, leaving a surface with higher chrome and nickel content. I have seen passivation called out on drawings for titanium and cobalt chrome parts. However, it’s often unclear why cobalt chrome or titanium would require passivation. One suggested reason is that exogenous iron could be introduced to the product by CNC cutting tools. This may have had some validity 60 years ago, when high speed steel cutting tools were used. Today’s cutting tools no longer contain any appreciable amounts of iron, and the risk of pick-up of exogenous iron is rare. Likely, the intent for passivation was related to cleaning rather than passivation. There are, however, valid reasons to passivate cobalt chrome and titanium, for instance, if the product is shot peened using stainless steel shot. Typical stainless steel conditioned cut wire shot used in orthopedics contains upwards of 50% iron. Passivation may be justified under these circumstances. It’s critically important for development engineers to know and communicate clearly the requirements and acceptance criteria for special processes.
#4) Correctly Define Inspection Datum Structure and Gauging Requirements
As orthopedic implant and instrument designs become more complex, we cannot overstate the importance of inspection and datum structures. First and foremost, datum structures should be defined based on the function of the device. Careful attention should be given to establishing datums on features. Only create necessary datums. Not all feature frames need three datums! In the absence of a functional need, the datum structure should be based on a combination of manufacturing capability and gauging/inspection. This is often a point of much discussion and disagreement. Involve subject matter experts when the decision is unclear. Invest time early in the development process to avoid issues during capability runs and production.
#5) Cautiously Leverage Previous Designs
Many engineers started their careers in medical devices. I did not. I did, however, develop products in highly regulated industrial and utility industries. The cost of a single failure of these products in lives and dollars was staggering. When I entered the medical device industry almost 20 years ago, I was somewhat surprised at the “design it, test it, make it, sell it” approach. Often, it seemed that design features and outputs were not clearly understood and less than optimal for performance and reliability. Today, design engineers leverage previous designs with good intentions only to find that many times, it was the supplier or manufacturer that would “compensate” to make the design work. Some refer to this as “tribal knowledge.” If you don’t have the tribal knowledge, the product may not function even if all the parts conform to specifications. Advice for today? Be cautious of what was done in the past. When leveraging previous designs, product engineers should invest time in reviewing current manufacturing practice, inspection methods, rejection rates and complaints.
#6) Use DF(x)
DFM, design for manufacturability, is well-known. DFM narrowly focuses on manufacturing. DF(x) greatly expands the perspective by taking into consideration all user need sources. DF(x) user needs are based on all inputs, not only from the customer, but from all functions of the business. Documenting these needs guides the development process and can be a critical factor in selecting design alternatives.
#7) Solicit Independent Expertise
My son is a medical device engineer. He has a great education, BS and MS in mechanical engineering and an MBA. My advice to him? “You will learn by making your own mistakes, or from someone else who has made mistakes.” I think he may have learned quite a bit from me, as I have made plenty of mistakes. There are many “experienced” engineers, inspectors and machinists who are more than willing to give their opinion. Engineers are advised to consider the advice of those with experience.
Often grumpy, often old
Many stories, they have told
Listen youngster to what they say
Lest you learn the hard way
#8) No Ambiguity
Every feature must be clearly defined. Every expectation of the engineer must be clearly communicated. I recall a design engineer who had a note on the drawing: grit blast, aluminum oxide 90-100 grit at 80 psi. When the parts arrived, he rejected them. He said they were not rough enough. The supplier said that they did what was on the drawing. The engineer replied that they must have done something wrong, because it was not what he wanted. He said the prototypes that were made by a different supplier were made according to the note on the drawing and they were right. The engineer told the supplier to make them again and this time they needed to get it right, as any further issues would delay the launch. The engineer did not understand all the variables for grit blasting. He did not take into consideration the age of the blast media, distance from the surface, angle of blasting, etc. With good intentions, the engineer specified what should be done on the drawing, but he failed to define what was actually required.
It must also be clear what governs the design: the 2D drawing or the solid model, or a combination of the two? I’ve seen engineers round edges on the part model with a 0.25 mm radius. The 2D drawing doesn’t show the radius. The only requirement on the drawing is to “break sharp edges.” Parts are rejected by the engineer because the radius is too small, even though the block tolerance on the 2D drawing is +/- 0.2 mm. A radius of 0.05 mm is barely detectable. The engineer says that the block tolerance does not apply, and the supplier should have known better.
#9) Carefully Develop the Design Validation Protocol
FDA is clear about the requirement for design validation. 820.30(g) Design Controls states, “Design validation shall ensure that devices conform to defined user needs and intended uses and shall include testing of production units under actual or simulated use conditions.”2 This makes sense from a regulation and product viability compliance perspective. It would not make sense to develop and offer a product that does not adequately perform the function for which it was created! The challenge is how to do design validation correctly. It begins with clearly defining user needs. Is the product intended for global use? Have user needs been considered for the differences in patient anatomy? Asian patients differ from typical American patients. Not only do patients differ, but surgeons do, as well. The hands of the typical American orthopedic surgeon are larger than those of the typical Asian surgeon. I have heard many complaints from Chinese surgeons, both male and female, that instruments were difficult to use because they were designed only with the input of American surgeons.
The first step in successful design validation is understanding and documenting clear user needs. The second step, defining the design validation protocol, is often the most challenging. Who should participate? How many participants are needed? How many samples/tests should be evaluated by each participant. What sizes should be evaluated? Do the participants adequately represent the scope of the market in their patient base and application/indication of the product? These are all critical questions that need to be addressed for successful design validation.
These nine steps have helped me decrease product development setbacks over the years. Certainly, more rules can be added to this list. What rules are on your list?
References
1. Code of Federal Regulations Title 21 Volume 8, Part 820, § 820.75 Process Validation, Food and Drug Administration, Department of Health and Human Services, 2019.
2. Code of Federal Regulations Title 21 Volume 8, Part 820, § 820.30 Design Controls, Food and Drug Administration, Department of Health and Human Services, 2019.
Dale Tempco is a consultant specializing in product development, operations, quality, supply chain, M&A and finance with expertise in low cost sourcing, design transfer, design for manufacturability, special process validation, cleaning validation, additive manufacturing, failure analysis, cost accounting and extensive experience in China.